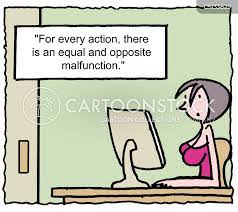
Introduction
Some things are easier to learn than others. Piaget, one of the fathers of the constructivist movement, talked about two types of learning: assimilation and accommodation (Atherton, 2009). Assimilation and accommodation are the two complementary processes through which awareness of the outside world is internalized (Atherton, 2009).
In assimilation, the perceptions of the outside world are incorporated into the internal world model without changing the structure of that model, but potentially at the cost of “squeezing” the external perceptions to fit (Atherton, 2009). In accommodation, the internal world model has to accommodate itself to the evidence with which it is confronted and thus adapt to it, which can be a more difficult and painful process (Atherton, 2009). Accommodation has everything to do with conceptual change, and is the starting point of the many theories concerned with misconceptions.
Constructivism tells us that learning has a lot to do with previous knowledge and experiences. A teacher must account for these preconceptions if she wants to influence the learning process. Most of the time, preconceptions are useful and, if accessed properly by the student, can help the student understand new concepts and fit them into the bigger picture, creating a shortcut to the new knowledge. However, some preconceptions are incorrect and there may be great challenges in overcoming these misconceptions with correct knowledge. The process of modifying these naïve misconceptions into scientifically acceptable concepts is called conceptual change (diSessa, 2006). This paper discusses conceptual change applied to teaching and learning about physical scientific principles; specifically learning about forces.
Learning scientists noticed there were many similar types of physics related misconceptions popping up in every classroom of the world. They are so universal that they seem a natural step through which most people pass. Researchers started recording the different types of misconceptions and, after over two decades of work and 3000 articles (Chi, 2005), researchers had a solid foundation for conceptual change research. The naïve conceptions were readily observed, but were extremely hard to modify. Researchers started theorizing about how these misconceptions were organized by the student, and why they were so common and resistant to change. Were they all connected in a consistent network of conceptions or were they just disorganized fragments that only became connected when formal teaching of physics began? Perhaps they were simply caused by misunderstood terms; or were they evidence of an existing intuitive, but incorrect, model?
These questions are very relevant to learning theory and especially the practicality of teaching physics. Depending on the type of classification of the misconceptions, a physics teacher should approach teaching these conceptions in different ways. For instance, if the problem lies in the misalignment of the word “force” the teacher should focus on explaining to the students that their language is what’s causing all the difficulty, and that their conception of force is actually momentum. If, on the other hand, the misconception is on a deeper level, and the idea of force for the novice is ingrained in a form of a complex knowledge system consisting of a network of beliefs and presuppositions (Vosniadou, 2002), then the teacher must undermine the whole system, which could be a much larger and longer effort. Therefore, it is important to understand the organization of these misconceptions.
Unfortunately there is no overall consensus on this topic in the learning sciences community. Many studies have been done to try to confirm or negate each theory of misconception structure, but this issue is tough to measure. In one case, diSessa, Gillespie and Esterly (2004) tried to duplicate an experiment by Ioannides and Vosniadou (2002) and were unsuccessful in repeating similar results. The following section is a review of some of the main theories of misconceptions and a few methodological attempts of conceptual change.
Literature Review
Theories of Misconceptions
Medieval and Intuitive Beliefs
Historically, the scientific understanding of force and how things move has evolved. Nersessian and Resnik (1989) explore the parallels between historical pre-Newtonian explanations of motion and those used intuitively by students and even adults. In the article, the authors develop an extensive model of the medieval belief structure and conceptual structure of motion and then compare the many studies investigating misconceptions of motions of intuitive physics. They sum up historical beliefs of motion into six main misconceptions (Nersessian & Resnik, 1989):
1) All motion requires a causal explanation.
2) Motion is caused by a mover.
3) Continuing motions is sustained by impetus (impetus – a stored-up force that is the property of the object, imparted to it by the mover).
4) Carrying does not convey impetus.
5) Downward motion is natural.
6) Heavier objects fall faster.
They then compiled a list of conceptions of naive physics about motion from the numerous studies of the 1970 and 1980. The authors notice that the naive conceptions about motion are almost identical to the beliefs gathered from historical records, calling them intuitive beliefs, because they come naturally to so many of us, without training, by self-explaining the world around us.
An important difference between intuitive beliefs and the Newtonian view is that all motion requires an explanation, while Newton states that motion, or constant velocity, is a state and that only a change of state, or acceleration, requires an explanation. Nersessian and Resnik (1989) also propose a conceptual structure of medieval theory of motion, linking all the beliefs together, and then hypothesized on the possible structure of intuitive physics. They demonstrate the striking similarity between intuitive and medieval conceptual / belief structures. Therefore, by comparing intuitive physics with historical pre-Newtonian physics, Nersessian and Resnik (1989) confirm that there could be an underlying structure of naive conceptions that generates intuitive explanations of certain types of motions.
Framework Theory
Vosniadou (2002) also rationalizes that the force and motion misconceptions are organized in a form of a coherent yet a narrow sort of internal theory. Vosniadou chooses to use the word framework as opposed to theory to describe her view of the organization of preconceptions in order to separate these internal, quasi-coherent explanatory systems from real scientific theories. Vosniadou (2002) believes conceptual change takes a long time and is a difficult process as it requires undermining an array of intuitive beliefs that are deep-seeded along with their complex interconnections. These frameworks act as constraints on the way individuals interpret environmental inputs to conjecture about the physical world. Specific theories formed through this process are continuously enriched and modified. Some kinds of conceptual change require the simple addition of new information to an existing conceptual structure. Others can only be accomplished when existing beliefs and presuppositions are revised.
An empirical study (Ionnides & Vosniadou, 2002) tries to probe the initial knowledge structures for the concepts of force and motion of children ranging from preschool to high school. The authors’ aim is to empirically support Vosniadou’s Framework theory. The results appear to support the Framework theory and that most of the children made use of a small number of relatively well-defined and internally consistent interpretations of force (Ionnides & Vosniadou, 2002). Also this cross sectional study gives rise to a progression of the different intuitive beliefs about motion. In particular, it seems that in their earlier years, children see force as an internal property of physical objects related to their weight. Then they metamorphose their view of force to be an acquired property of objects, similar to the impetus idea from historical accounts. Finally, when formal teaching of physics begins at school (around the age of 15), students start combining several different meanings of force, trying to assimilate the notions of “gravity” and “force of push and pull” into their already set framework. This creates confusion in the internal model of force and motion and thus leads to increased fragmentation and a less cohesive framework (Ionnides & Vosniadou, 2002).
Force as a Property of a Substance
Alternatively, Reiner, Slotta, Chi and Resnik (2000) see misconceptions arising when people use their understanding of substances to explain motion and force. From their daily lives and their observations, people learn about objects and substances in general. They make connections and eventually create an internalized model of how substances behave; specifically, substances are pushable, are frictional, are able to be contained, can be consumed, have definite location, are able to move or be moved, are stable, have surface and volume, require force to move, and fall down when dropped (Reiner et al, 2000). Consequently misconceptions arise on the ontological level with a scientific concept such as force.
The authors (Reiner et al, 2000) propose that intuitively understood force is associated with a substance ontology when in fact these two concepts belong to a process ontology; the novice sees force as a property of substances, instead of a process, making sense of the concept of force by appealing to their reliable understanding of the material world through a substance schema. In the case of the impetus misconception (described by Nersessian & Resnik, 1989), students often believe that the impetus object exerts a force on another material object, thus providing it with the potential to exert a force on a third object. This is consistent with the belief that the impetus-force is an extensive property of the first object, which may be transferred to the second, and then onto the third, or even that the force is an actual substance that is carried along by the first object and then transferred over to the second (Reiner et al, 2000). Therefore force is not considered a process of interaction but instead either a substance or a property of a substance.
This misconception theory supports the claim that misconceptions are not fragmented but instead form an ontologically misaligned structure based on the substance intuitive model.
Direct versus Emergent Models of Force
In another more recent article (Chi, 2005), Chi changes her position on her view of misconceptions, and points to the evolution of her theory:
The current thesis has evolved over the last decade. Our prior analyses were incomplete in several ways and have metamorphosed several times. The metamorphosis is reflected in the various names we have used (many incorrectly) over the years to refer to emergent processes, ranging from “events” (Chi, 1992), to “acausal interaction processes” (Chi, 1993; Chi & Slotta, 1993), to “constraint-based interactions” (Chi, de Leeuw, et al., 1994; Slotta, Chi & Joram, 1995; Slotta & Chi, 1996), to “equilibration processes” (Chi, 1997a; Ferrari & Chi, 1998), to “complicated, abstract and dynamic concepts” (Chi, 2000a), and to “complex, dynamic processes” (Chi&Roscoe, 2002).The evolution of names reflects our improved understanding. More recently, we have used these ideas to discriminate between robust and non-robust misconceptions (Chi&Roscoe, 2002), as well as to apply some of these ideas to scientific discoveries (Chi & Hausmann, 2003). This article represents a more complete but still evolving exposition of this theoretical explanation. (Chi, 2005, pp 164)
In her 2005 version, Chi theorizes about why some misconceptions are so hard to change, calling them robust. She begins by explaining that in science there is a distinction between direct processes and emergent ones. Relations between objects produce emergent behaviours that are not apparent in the description of either the object or the relation (Leher & Schuable, 2006). For instance, the “V” shape of a bird flock is emergent: The birds fly according to what is most aerodynamically efficient for each individual bird. Flying behind and to the side of a bird is what is easiest for each bird, and the V shape is what emerges when all these individual birds do the similar thing. The bird is the component of the process; the pattern is the V shape that emerges.
Chi (2005) explains the difference between emergent and direct processes using two different flow mechanisms: circulation being a direct process and diffusion an emergent one. Direct and emergent processes are very different on the constituent level: In a direct process every element has distinct behaviours, has constrained interactions, is sequential, is dependent on each other, has terminating interactions, is said to be part of a subgroup or class, directly affects the pattern, corresponds to the overall pattern, and has global goal or is intentional. While in an emergent process every element behaves uniformly, is unconstrained, has simultaneous interactions, is independent of other elements, has continuous interactions, has non-direct effect on the overall pattern, has disjointed behaviour from the overall pattern, and has only local goals or unintentional (Chi, 2005).
When Chi examined several responses of students about how diffusion occurs, it was evident that the students’ explanations contained many attributes of a direct process rather than of an emergent one. Chi speculates that this would be the case in terms of any emergent process, with forces and motion included. She believes that students’ commonsense ideas of process correspond more closely to a direct kind rather than emergent. They rely on their commonsense understanding of direct processes to interpret emergent ones causing these robust misconceptions (Chi, 2005). Although a very compelling theory, Chi realizes that there is yet not enough empirical data to support her thesis.
Knowledge in Pieces
On the other end of the spectrum is the theory of Knowledge in Pieces (diSessa, 1993). This view envisions that intuitive physics is made up mostly of hundreds or thousands of self-explanatory schemata, typically abstracted from common situations, called phenomenological primitives (p-prims). P-prims are loosely organized, and sometimes highly contextual, so that the word “theory” is inappropriate (diSessa, 2006). DiSessa argues that intuitive thinking is not developed sufficiently to constitute a theory, yet her opponents believe that intuitive thinking about motion can still have a structure and consistency across contexts (Vosniadou, 2002). To deny this claim, diSessa and her colleagues (diSessa, Gillespie, & Esterly, 2004) set out to replicate Ionannides and Vosniadou’s empirical study (2002), and to extend it to show the incompleteness of the original study. The authors took great care to discuss the theoretical issues of misconceptions and define the terminology used.
First, they explicitly state that context is the central concept in the debate between advocates of coherence versus fragmentation. After an extensive discussion of context and fragmentation, the authors present a new term to describe their model: Crowded-irregular knowledge systems entail many elements, overlapping contexts that are ad hoc in their specification, and have numerous instabilities(diSessa, Gillespie, & Esterly, 2004). The other relevant concept diSessa, Gillespie, & Esterly (2004) point to is specificity of an intuitive belief or misconception. They notice that in the Ionnides and Vosniadou’s study (2002) misconceptions were only recorded on the basis of existential aspect (i.e. answering the question: Does a force exist?). But what about the quantitative aspect (i.e. How much force in comparison is there?), the ontological aspect (i.e. What is the nature of the force?), the compositional aspect (i.e. Do forces combine or act on each other?), or even the causal aspect (i.e. What are the consequences of the existence of a force?). Hence, the authors decided to extend the original design of Ionannides and Vosniadou’s study to investigate more of these specifications.
DiSessa, Gillespie, & Esterly (2004) were not able to replicate the results of the original study. In fact, students in the study didn’t seem to have a “small number of relatively well-defined and internally consistent interpretations of force” as was the thesis of Ionannides and Vosniadou (2002). Moreover, their extension study, taking into account context and specificity, demonstrated that the concept of force is very diverse among different students and it doesn’t seem to be internally consistent as proposed by Vosniadou (2002).
Conceptual Change Methodology
Given the possible ways misconceptions are organized in the naïve physicists mind determines the appropriate methodology of instruction. If, for instance, we assume that diSessa is correct in saying that all knowledge is divided into numerous p-primes then the way to teach physics is to organize and systematize all of these fragments into something that resembles Newtonian mechanics (diSessa, 1993). On the other hand, if we assume that Vosniadou’s Framework Theory is more correct, we would have to undermine the whole framework and replace it slowly with the scientifically appropriate theory. Vosniadou (2002) argues that conceptual change does not happen suddenly, because we are dealing with a complex knowledge system that consists of a network of beliefs or presuppositions that take a long time to change. But if we assume Reimer’s, Slotta’s, Chi’s and Resnik (2000) perspective that the misconceptions are ontological in nature and that physicist neophytes see force as a property of a substance instead of a process, then the shift to the correct understanding would be abrupt, radical and would occur in a short amount of time. Finally, if we see the misconceptions as Chi’s (2005) most recent hypothesis, where the intuitive beliefs are ontologically misclassified, a re-representation or a conceptual shift across ontological kinds needs to occur. In this case, misconceptions of the emergent kind are robust (and therefore hard to modify) and such a shift requires that students know about the emergent nature of force and can overcome their predisposition to conceive of all processes as direct.
Therefore, depending on the theory assumed about misconceptions, the approach to overcome them should be different. However, while the debate rages on about the nature of misconceptions, and teachers still attempt to produce conceptual change in students. The following three studies show three different strategies that are very accessible to the average teacher. They require few resources and could be an addition (or partial replacement) to the traditional classroom. Small changes, as opposed to huge transformations of the classroom, are more realistic and are more likely to be adopted by teachers.
CSCL in the Physics Classroom
As an example, Tao and Gunstone (1999) used a computer-supported collaborative learning environment (CSCL) to undertake conceptual change in force and motion. Their research question was: (a) How effective is conceptual conflict in fostering conceptual change? (b) Is conceptual change realized as a development (addition), a replacement of alternative conceptions, the coexistence of a range of conceptions with each coming into play in a specific context, or some other process? Even though in their research questions there was no mention of CSCL, their method was primarily to use simulations and collaborative learning to confront student’s conceptions. The assumption was that once students realized their original conception doesn’t match with what actually happens physically, the student will reconsider their own beliefs and possibly accept the scientifically correct version.
In the field study, students were grouped into pairs, and over a span of 10 weeks they worked with a computer simulation program called the Force and Motion Microworld. Each task required the students to: (1) Make a prediction about the consequences when certain changes were made to the program. (2) Explain their prediction. (3) Run the program to test their prediction. (4) Reconcile any discrepancy between their prediction and the observation in the Microworld. Even though students were asked explicitly to predict what they would see, and then reflect on what they actually saw in the simulation, thus confronting their misconceptions, this didn’t reflect in their reactions. The authors didn’t observe any sighs of amazement or outbursts of conflict. It’s as though they were going through the motions of the procedure of the CSCL activities but didn’t understand nor care to delve deeper into the situation. It is therefore no surprise that few students increased their conceptual understanding of force and motion (decreasing their misconceptions).
Through post study interviews with the students, Tao and Gunstone realized that some of the students had no opportunity to reflect on the conflict they encountered in the simulations due to ill-formed partnerships. In the interview, when confronted with the conflict, the students realized that their conception was false, and changed some of their ideas of force and motion. The researchers therefore concluded that conceptual conflicts were not enough to produce conceptual change. They asserted that cognitive engagement is an important factor: “For conflicts to lead to change, students need to reflect on and reconstruct their conceptions.” (Tao & Gunstone, 1999, pp 870).
Another interesting observation in the Tao and Gunstone (1999) study was that the misconceptions were very much context based. Three situations (and simulations) of the same physical principal were presented: one of a model car, one of a spaceship, one of a skydiver. After the CSCL intervention, the students were asked to discuss each situation. Even though physically the three situations were identical, more students had misconceptions about the model car situation as opposed to the spaceship and skydiver. The authors speculated that this was due to the students’ familiarity of the car situation, thus the misconception being more profound and harder to change, yet the spaceship and the parachutist contexts were relatively new and not as developed, thus easier to change.
Finally, Tao and Gunstone (1999) also noted that for the students that were not at all motivated during the CSCL activity, absolutely no conceptual change occurred. Being off task, not motivated to participate in the collaborations with their partner, these students’ prior conceptions remained untouched. Motivation, therefore, seems to be one of the most important indicators of whether any conceptual change will occur.
Class Discussions to Promote Conceptual Change
Eryilmaz (2002) used a different approach to produce conceptual change. He wanted to see what effect conceptual assignments and conceptual change discussions had on students’ misconceptions of force and motion. Comparing traditional physics teaching versus regular teaching with inserted conceptual assignments and follow up classroom discussions on conceptual change, he found that the discussions were very advantageous in terms of producing conceptual change.
Eryilmaz took a quantitative approach in his research, studying close to 400 students from 18 physics classes. Over a span of a term, half the teachers were to use their regular methods of teaching, and the other were instructed to use classroom discussions on conceptual change at least once a week. To control for the type of discussions occurring in the 9 separate classrooms, the teachers received training and guidelines to keep the discussions consistent over the many teachers participating. As in Tao and Gunstone’s CSCL activity, Eryilmaz focused on conceptual conflict to attempt conceptual change. The core of the discussion was for students (1) to understand their own beliefs and conceptions about force and motion; (2) create a discrepant event, one that creates conflict between exposed preconceptions and some observed phenomenon that students cannot explain – let students become aware of this conflict; (3) let students reflect upon the conflict and show explicitly where and how their preconceptions and the accepted force and motion theories differed.
In the end, the students from the conceptual change discussion classes and conceptual assignment classes outperformed the students from the traditional classes with respect to the reduction of force and motion misconceptions. Although significantly different from traditional teaching, the difference in lowering misconceptions from start to finish of the intervention was small (small effect size). This once again demonstrates how resistant misconceptions are to change.
Teaching by Example
In yet another attempt to produce conceptual change, a study compared types of examples and the way they were used in teaching (Brown, 1992). In this study, students were presented with two different types of text explaining Newton’s Third Law (the law of action and reaction). The first explanation was basically a statement of Newton’s Third Law followed by many examples of where and how it is applied, from pushing a finger against a rock, to legs pushing against the floor while walking, to recoil while shooting a gun, and finally to the specific example where a book is sitting on a table with the table exerting a force on the book. This type of explanation uses induction from examples to generate an understanding for Newton’s Third Law, which in turn should be applied to new situations (the book on the table). The second text also stated Newton’s Third Law, but then attempted to take the reader through a journey from a hand pushing on a spring and the spring pushing back on the hand (an anchoring situation), through progressively more similar situations to the final outcome of the book sitting on the table having a force being pushed up onto it (the target problem). Brown called this a bridging explanation. Within this bridging explanation, a mechanistic model was developed for the reader (compressing and/or bending of rigid objects on a microscopic scale) which now could be applied in a general sense.
The results of this research show the power of the anchoring analogy followed by the bridging explanations in producing conceptual change. Not only did the students reading the second text overwhelmingly outperform on a follow-up misconceptions questionnaire with alternate contexts of Newton’s Third, they also scored extremely high on “how much their answers made sense”, as compared to the students that read the induction from multiple examples type text (Brown, 1992).
This study supports Chi’s (2005) recent theory on misconception organization, in the sense that the force and motion are ontologically misclassified as being of the direct nature. But if an emergent model of force is undeniably illustrated, conceptual change will happen radically and in a short amount of time. Unfortunately, this study investigated only a small amount of participants (only 14 students with the initial misconception of the table not pushing up on the book received the two different text explanations) and did not investigate long-term effects of the bridging explanation on conceptual change. However, it seems that the method of anchoring analogy followed by bridging explanations have great potential in influencing conceptual change and overcoming misconceptions in novice physicists.
Discussion and Conclusion
From the three presented methods of conceptual change, it appears that the best option was Brown’s (1992) anchoring analogy followed by the bridging explanation. The other two seemed to follow the Piaget’s idea of accommodation, yet they only had a slightly better effect at producing conceptual change as compared to traditional teaching. This, I believe, is because Brown’s method (1992) tackled the underlying problem with these robust misconceptions: The mechanistic model gave a way for students to understand that force is not a direct process, but instead an emergent one. Once students understood the mechanistic model, their understanding of what is a force changed in an ontological way. They had a way to generalize each different situation, explain it with their model, and their naïve misconceptions were instantly replaced. This process was radical and occurred in a short period of time.
Since Brown’s (1992) study was prior to Chi’s (2005) ontological redefinition of her direct versus emergent model of robust misconceptions, he did not include her idea as part of his theoretical backing. But if Brown was to redo his study now after Chi’s interpretation, I am certain he would have made the connection.
The other two methods of conceptual change (Eryilmaz, 2002; Tao & Gunstone, 1999) were both interested in confronting students with discrepant events that contradict their existing conceptions to produce disequilibration, as recommended by Piaget (Atherton, 2009). This, in theory, induces students to reflect on their conceptions as they try to resolve their conflict. I believe that this disequilibrium only works in a given context, possibly without transferring to other situations, and for sure without generalization. For instance a spaceship travelling through space with no force acting on it would continue travelling at the same rate, but in Tao & Gunstone’s study (1999), this idea did not transfer to the model car situation. Students didn’t see these two situations as the same: One is on earth – one is in space; one has an engine – the other does not; one is usually seen in a frictional environment, with model cars usually stopping after a set period of time, the other is set in space with no resistance.
Using conflict to produce conceptual change therefore seems fruitless if we want the students to see a generalization of force and motion. We are not giving the students a model to replace their own. Instead we are telling them that they were wrong, and this is what actually happens in this particular situation. Since an alternative to their own explanation is not presented, their misconceptions will remain if only slightly modified. We assume students can see the similarity between contexts, as physicists do, but I believe, as does diSessa (diSessa 1993; diSessa 2006; diSessa,Gillespie, & Esterly, 2004) that this assumption is wrong. Until the students have a generalizable model that makes sense to them and is applicable to every type of situation of motion and force, conceptual change by confrontation and disequilibration is futile, only confronting one p-prim at a time.
I, therefore, offer a slightly modified version and combination of a theory of misconceptions. Evidently, when novices encounter physics phenomena, initially, students have a very context specific view. I hypothesize that this is because intuitively they see force and motion as direct processes and not emergent as they are. If force was a direct process, then possibly the situations presented and assumed to be equivalent would actually be different, not just in terms of context, but in terms of the processes. Therefore the wrong ontology (Chi, 2005) of force and motion makes the observer see the physical world as a huge array of many situations that have little to do with each other (Knowledge in Pieces: diSessa, 1993). However, the moment the person realizes the underlying structure at the molecular level and understands the emergent model of force, they will see how all the different fragmented phenomena are disguised versions of the same concept, as it happened in Brown’s study (1992).
In all the articles I read, diSessa’s fragmented theory and Chi’s ontological mis-categorization theory are always presented on opposite ends of the misconception theory spectrum. I, however, see these two theories complementary: one explaining the other.
References
Atherton, J. S. (2009) Learning and Teaching; Assimilation and Accommodation [On-line] UK: Available: http://www.learningandteaching.info/learning/assimacc.htm Accessed: 10 December 2009
Brown, D. E. (1992). Using Examples and Analogies to Remediate Misconception in Physics: Factors
Influencing Conceptual Change. Journal of Research in Science Teaching, 29(1), 17-34.
Chi, M. T. H. (2005). Commonsense Conceptions of Emergent Processes: Why Some Misconceptions
Are Robust. Journal of the Learning Sciences, 14(2), 161-199.
diSessa, A. (1993). Toward an Epistemology of Physics. Cognition and Instruction, 10, 105-225
diSessa, A. A. (2006). A history of conceptual change research: threads and fault lines. In. K. Sawyer
(Ed.), The Cambridge Handbook of the Learning Sciences (pp. 265-282). MA: Cambridge University Press.
diSessa, A. A., Gillespie, N., & Esterly, J. (2004). Coherence vs. Fragmentation in the Development of
the Concept of Force. Cognitive Science, 28, 843-900.
Gopnik, A., & Wellman, H. M. (1994). The Theory Theory. In L. A. Hirschfeld & S. A. Gelman (Eds.),
Mapping the Mind: Domain Specificity in Cognition and Culture (pp. 257-293). New York:
Cambridge University Press.
Eryilmaz, A. (2002). Effects of Conceptual Assignments and Conceptual Change Discussions on
Students’ Misconceptions and Achievement Regarding Force and Motion. Journal of Research
in Science Teaching, 39(10), 1001-1015.
Ioannides, C. & Vosniadou, C. (2002). The Changing Meanings of Force. Cognitive Science
Quarterly, 2, 5-61.
Lehrer, R., & Schauble, L. (2006). Cultivating Model-Based Reasoning in Science Education. In. K.
Sawyer (Ed.), The Cambridge Handbook of the Learning Sciences(pp. 371-388). MA:
Cambridge University Press.
Neressian, N. J., & Resnick, L. B. (1989). Comparing Historical and Intuitive Explanations of Motion:
Does “Naive Physics” Have a Structure? Proceedings of the 11th Annual Conference of the
Cognitive Science Society (pp. 412-417). Hillsdale, NJ: Lawrence Erlbaum Associates.
Reiner, M., Slotta, J. D., Chi, M. T. H., & Resnick, L. B. (2000). Naive Physics Reasoning: A
Commitment to Substance-Based Conceptions. Cognition and Instruction, 18(1), 1-34.
Stahl, G., Koschmann, T. & Suthers, D.D. (2006). Computer-Supported Collaborative Learning. In. K. Sawyer
(Ed.), The Cambridge Handbook of the Learning Sciences (pp. 409-426). MA: Cambridge University Press.
Tao, P.-K., & Gunstone, R. F. (1999). The Process of Conceptual Change in Force and Motion during
Computer-Supported Physics Instruction. Journal of Research in Science Teaching, 36(7),
859-882.
Vosniadou, S. (2002). M. Limon & L. Mason (Eds.), Reconsidering Conceptual Change, Issues in
Thoery and Practice (pp. 61-76). Kluwer Academic Publishers.